Fundamentals of Fluid Flow in Porous Media
Chapter 5
Miscible Displacement
Fluid Properties in Miscible Displacement: Macroscopic Displacement Efficiency
Optimization of Vertical Miscible Flood Performance Through Cyclic Pressure Pulsing[1]
The study of this method has been done on the Rainbow Lake reefs oil of Northern Alberta. In this field oil was displaced downward by a less viscous solvent than was injected as a slug followed by chase gas. The injection flow rate was high enough that the solvent front velocity in the formation be higher than the critical velocity for a stable front displacement so viscous fingering, which was in part triggered by local heterogeneities, was blamed for reduced sweep efficiencies and oil recoveries which were less than what was expected in the design stage. Viscous fingering leads to soon breakthrough of the solvent and chase gas. When both solvent and chase gas break through, the gas to oil ratio (GOR) increases dramatically with a comparable decrease in oil production.
The standard approach in controlling excessive gas or solvent production is work overs in which the current perforations are cement squeezed and new perforations are made lower in the reef. This procedure is costly and is not always successful. Husky proposed another procedure in optimizing the displacement strategy which an improve the vertically directed miscible floods, by applying a cyclic flow interruption consisting of periodical shut-in. Experimental and numerical results showed that the concept of shut-in leads to significant incremental oil recoveries. Indeed during the shut in period solvent and oil have time to settle under gravity effect and there is an extra time for mass transfer between solvent and oil.
The problem with the cyclic flow interruptions is that production is discontinued. The question was raised whether a similar effect could be observed through a continuous process.
It was proposed that cyclic pressure pulsing under restricted flow could increase mass transfer between solvent and oil and also force recovery from dendritic structures or other upswept areas through pressure pulsing of the solvent and gas phases. Kantzas et al. ran a series of vertical miscible flood tests on a core from the Rainbow Keg River area. All runs were performed at rates significantly higher that the critical rate for the specific system. Methane was used as the solvent and n-pentane as the oil. The choice of materials (methane and n-pentane) was made to match density and viscosity rations of the reservoir fluids while maintaining a simple composition of each phase, so that effluent analysis could be simplified. Figure 5‑42 shows their laboratory apparatus. The core was always mounted in the vertical position and saturated with n-pentane. Methane was injected at various rates from the top of the core. Different pressure pulsing scenarios were also applied. Sixteen experiments were discussed in their report. For each experiment at different time steps recovery, cumulative recovery, GOR and mass fraction of each fluid in the effluent using gas chromatograph data and a Peng-Robinson type algorithm.
The base case that is used to evaluate other process is continuous injection of the solvent. Injection rate was approximately ten times of the critical rate. To simulate the shut in process, the same rate was maintained, but every two hours the experiment was shut in for a day. The shut in test lasted for around 75 hours. The incremental recovery compare to the base case (continuous injection) was around 6% OOIP. So shut in can provide significant incremental oil recovery with zero incremental investment. However, since the project is extended for significantly longer periods of time, the return on the initial investment may be perceived as unattractive.
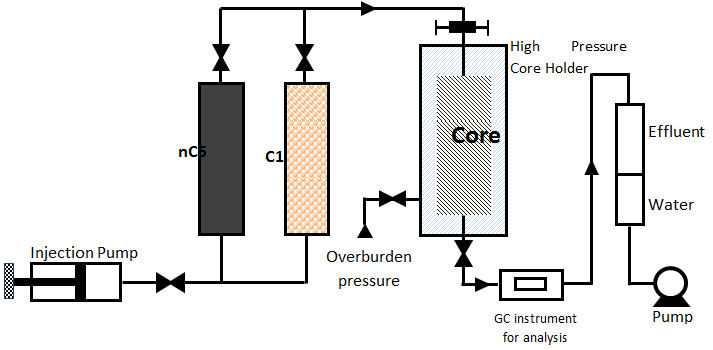
Figure 5-42: Schematic of the Experimental Apparatus
Two groups of the tests were designed to study the shut in and pressure pulsing scenario. In the first group pressure pulsing and shut-in scenarios were applied with the assumption that the average rates have to be maintained at the same value, 10 times of the critical rate. The second group of experiments consisted of a combination of rate reduction tests accompanied by pressure pulsing / shut-in cycles. The pressure pulse was achieved by pressurizing the injection cylinder to approximately 2,000 kPa over the normal operating pressure while the production was shut-in. When the high limit pressure was reached, the injection was stopped and production resumed until the pressure had dropped approximately 2,000 kPa lower than the normal operating pressure (i.e. a pressure cycle of 4,000 kPa). At this point the pressure cycle was repeated. Since the pressure pulsing scenarios are evaluated for mature miscible floods, pressure pulses always started after solvent breakthrough.
It was found that with respect to rate reductions reducing the rate by half does not increase the recovery of oil. However, rate reduction after breakthrough gave a recovery increase of approximately 3% OOIP. Reducing the rate and applying pressure pulsing at the same time also provides better recoveries than the base case. Up to 4% incremental recovery of OOIP can be obtained.
Figure 5‑43 is the conventional plotting of mole fraction of solvent in effluent as a function of pore volumes injected. As it can be seen, after every shut-in period the methane mole fraction drops, the lower the amount of pentane left in the core, the lower the drop in the methane mole fraction. This can be explained by the fact that bypassed n-pentane is allowed to mix and/or flow towards the production end, this increasing the ultimate recovery.
The plots for the base case compared to a combination of pressure pulsing with rate reduction are shown in Figure 5‑44. In this figure the recovery plot as a function of pore volumes of solvent injected.
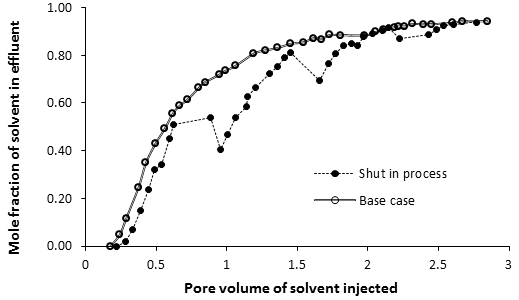
Figure 5-43: Plot of Mole Fraction of Solvent in the Effluent as a Function of the Pore Volumes of Solvent Injected. Periodic Shut-in Case
Each of the pressure pulses is shown in the stepwise increase of production. The horizontal part of the step is the pressure buildup, while the vertical part of the step is the pressure relief, where actually the incremental production occurs.
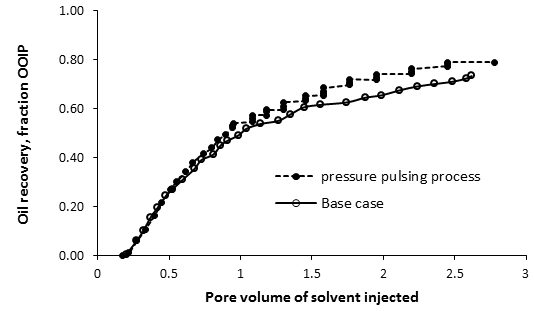
Figure 5-44: Cumulative Recovery for Rate Reduction and Pressure Pulsing as a Function of Pore Volumes of Solvent Injected
The plot of the mole fraction of solvent in the effluent is shown in Figure 5‑45. The response is similar to Figure 5‑43. However, the reduction in solvent mole fraction is significantly lower in the pressure pulsing case, than in the shut-in case, indicating that the pressure pulse occurred too fast and appreciable mixing did not take place. The combination of flow reduction with pressure pulsing shown in Figure 5‑44 provide the incremental recovery without any increase in GOR. From this point of view pressure pulsing accompanied by rate reduction can be considered as a tool for better reservoir management and reduction of operating costs. The combination of shut in and pressure pulsing process showed around 11% incremental recovery compare to the base case.
According to the Kantzas et al. experimental results it could be concluded that the life of a mature vertical miscible flood can be extended if the miscibility is enhanced. Such an enhancement can be achieved through periodic flow interruptions (shut-in), or rate reduction combined with cyclic pressure pulsing.
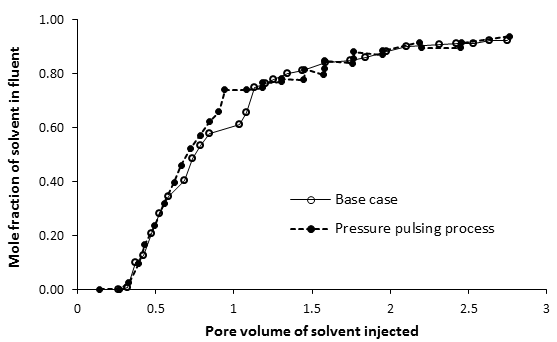
Figure 5-45: Mole Fraction of Solvent in the Effluent as a Function of the Pore Volumes of Solvent Injected. Rate Reduction and Pressure Pulsing Case
References
[1] “Optimization of Vertical Miscible Flood Performance through Cyclic Pressure Pulsing”, Kantzas, A., Marentette, D.F., See, D., Adamache, I., Mclntyre, F.I., Sigmund, P.M., JCPT, vol. 33, July 1994
Questions?
If you have any questions at all, please feel free to ask PERM! We are here to help the community.
The study of this method has been done on the Rainbow Lake reefs oil of Northern Alberta. In this field oil was displaced downward by a less viscous solvent than was injected as a slug followed by chase gas. The injection flow rate was high enough that the solvent front velocity in the formation be higher than the critical velocity for a stable front displacement so viscous fingering, which was in part triggered by local heterogeneities, was blamed for reduced sweep efficiencies and oil recoveries which were less than what was expected in the design stage. Viscous fingering leads to soon breakthrough of the solvent and chase gas. When both solvent and chase gas break through, the gas to oil ratio (GOR) increases dramatically with a comparable decrease in oil production.
The standard approach in controlling excessive gas or solvent production is work overs in which the current perforations are cement squeezed and new perforations are made lower in the reef. This procedure is costly and is not always successful. Husky proposed another procedure in optimizing the displacement strategy which an improve the vertically directed miscible floods, by applying a cyclic flow interruption consisting of periodical shut-in. Experimental and numerical results showed that the concept of shut-in leads to significant incremental oil recoveries. Indeed during the shut in period solvent and oil have time to settle under gravity effect and there is an extra time for mass transfer between solvent and oil.
The problem with the cyclic flow interruptions is that production is discontinued. The question was raised whether a similar effect could be observed through a continuous process.
It was proposed that cyclic pressure pulsing under restricted flow could increase mass transfer between solvent and oil and also force recovery from dendritic structures or other upswept areas through pressure pulsing of the solvent and gas phases. Kantzas et al. ran a series of vertical miscible flood tests on a core from the Rainbow Keg River area. All runs were performed at rates significantly higher that the critical rate for the specific system. Methane was used as the solvent and n-pentane as the oil. The choice of materials (methane and n-pentane) was made to match density and viscosity rations of the reservoir fluids while maintaining a simple composition of each phase, so that effluent analysis could be simplified. Figure 5‑42 shows their laboratory apparatus. The core was always mounted in the vertical position and saturated with n-pentane. Methane was injected at various rates from the top of the core. Different pressure pulsing scenarios were also applied. Sixteen experiments were discussed in their report. For each experiment at different time steps recovery, cumulative recovery, GOR and mass fraction of each fluid in the effluent using gas chromatograph data and a Peng-Robinson type algorithm.
The base case that is used to evaluate other process is continuous injection of the solvent. Injection rate was approximately ten times of the critical rate. To simulate the shut in process, the same rate was maintained, but every two hours the experiment was shut in for a day. The shut in test lasted for around 75 hours. The incremental recovery compare to the base case (continuous injection) was around 6% OOIP. So shut in can provide significant incremental oil recovery with zero incremental investment. However, since the project is extended for significantly longer periods of time, the return on the initial investment may be perceived as unattractive.
Figure 5-42: Schematic of the Experimental Apparatus
Two groups of the tests were designed to study the shut in and pressure pulsing scenario. In the first group pressure pulsing and shut-in scenarios were applied with the assumption that the average rates have to be maintained at the same value, 10 times of the critical rate. The second group of experiments consisted of a combination of rate reduction tests accompanied by pressure pulsing / shut-in cycles. The pressure pulse was achieved by pressurizing the injection cylinder to approximately 2,000 kPa over the normal operating pressure while the production was shut-in. When the high limit pressure was reached, the injection was stopped and production resumed until the pressure had dropped approximately 2,000 kPa lower than the normal operating pressure (i.e. a pressure cycle of 4,000 kPa). At this point the pressure cycle was repeated. Since the pressure pulsing scenarios are evaluated for mature miscible floods, pressure pulses always started after solvent breakthrough.
It was found that with respect to rate reductions reducing the rate by half does not increase the recovery of oil. However, rate reduction after breakthrough gave a recovery increase of approximately 3% OOIP. Reducing the rate and applying pressure pulsing at the same time also provides better recoveries than the base case. Up to 4% incremental recovery of OOIP can be obtained.
Figure 5‑43 is the conventional plotting of mole fraction of solvent in effluent as a function of pore volumes injected. As it can be seen, after every shut-in period the methane mole fraction drops, the lower the amount of pentane left in the core, the lower the drop in the methane mole fraction. This can be explained by the fact that bypassed n-pentane is allowed to mix and/or flow towards the production end, this increasing the ultimate recovery.
The plots for the base case compared to a combination of pressure pulsing with rate reduction are shown in Figure 5‑44. In this figure the recovery plot as a function of pore volumes of solvent injected.
Figure 5-43: Plot of Mole Fraction of Solvent in the Effluent as a Function of the Pore Volumes of Solvent Injected. Periodic Shut-in Case
Each of the pressure pulses is shown in the stepwise increase of production. The horizontal part of the step is the pressure buildup, while the vertical part of the step is the pressure relief, where actually the incremental production occurs.
Figure 5-44: Cumulative Recovery for Rate Reduction and Pressure Pulsing as a Function of Pore Volumes of Solvent Injected
The plot of the mole fraction of solvent in the effluent is shown in Figure 5‑45. The response is similar to Figure 5‑43. However, the reduction in solvent mole fraction is significantly lower in the pressure pulsing case, than in the shut-in case, indicating that the pressure pulse occurred too fast and appreciable mixing did not take place. The combination of flow reduction with pressure pulsing shown in Figure 5‑44 provide the incremental recovery without any increase in GOR. From this point of view pressure pulsing accompanied by rate reduction can be considered as a tool for better reservoir management and reduction of operating costs. The combination of shut in and pressure pulsing process showed around 11% incremental recovery compare to the base case.
According to the Kantzas et al. experimental results it could be concluded that the life of a mature vertical miscible flood can be extended if the miscibility is enhanced. Such an enhancement can be achieved through periodic flow interruptions (shut-in), or rate reduction combined with cyclic pressure pulsing.
Figure 5-45: Mole Fraction of Solvent in the Effluent as a Function of the Pore Volumes of Solvent Injected. Rate Reduction and Pressure Pulsing Case
References
[1] “Optimization of Vertical Miscible Flood Performance through Cyclic Pressure Pulsing”, Kantzas, A., Marentette, D.F., See, D., Adamache, I., Mclntyre, F.I., Sigmund, P.M., JCPT, vol. 33, July 1994
Questions?
If you have any questions at all, please feel free to ask PERM! We are here to help the community.